
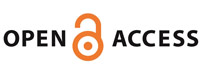
Summary
Lung cancer exhibits the highest mortality rate of all malignant tumors, and only a minority of non-small cell lung cancer (NSCLC) patients are diagnosed with localized, early stage tumors. Unfortunately, NSCLC is usually detected at advanced and inoperable stages. Tumor biomarkers can detect early stage lung cancer independently or in combination with low-dose computed tomography-based screening techniques. A liquid biopsy is a noninvasive modality for the pathological and molecular characterization of cancer, and it can be isolated from bodily fluids. There are a variety of biological elements that can be isolated from the peripheral blood, such as exosomes, circulating cell-free (tumor) DNA, circulating tumor cells, and microRNA. A liquid biopsy can be used to detect somatic mutations before treatment and dynamically during treatment, and to monitor the treatment response. It can also be used for minimal residual disease quantification, and to determine the emergence of therapy resistance. Despite the numerous research studies showing successful results, clinically available lung cancer biomarkers do not have sufficiently high specificity and sensitivity for widespread use. Therefore, universal experimental and therapeutic research regarding advanced molecular diagnostics has become necessary.Introduction
Lung cancer is one of the most frequently diagnosed cancers, and it is the number one cause of death due to cancer worldwide [1]. Non-small cell lung cancer (NSCLC) constitutes approximately 85% of all lung neoplasms. NSCLC patients with pathological stage IA tumors have a 5-year survival rate of 73%, while stages IIB and IIIB have rates of 36% and 9%, respectively. Unfortunately, the long-term lung cancer survival rate currently remains low, because the majority of the cases are detected at advanced and inoperable stages of the disease [2,3]. Even in cases of complete surgical resection, the 5-year survival probability is unsatisfactory [4,5]. Following patients with thorax computed tomography (CT) scans every 6 months for the first 2 to 3 years after a complete surgical resection has been recommended by the National Comprehensive Cancer Network guidelines [6]. However, recurrence or metastasis occurs in approximately 30%–70% of all complete resection cases [7]. The leading cause of death in cancer patients is metastasis, in which malignant cells spread the primary tumor to distant organs through the blood circulation via a multistep process [8]. A delayed diagnosis and the lack of reliable biomarkers are the main reasons for lung cancer detection in the advanced stages and its high mortality rates [9].Biological elements are secreted from cancer cells into their microenvironment in order to promote oncogenesis, and to protect themselves from the immune system [10]. A liquid biopsy is a noninvasive strategy for the pathological and molecular characterization of cancer, and it can be isolated from bodily fluids, such as the peripheral blood, cerebrospinal fluid, pleural fluid, urine, and saliva [11,12]. In this review, we will discuss the roles of liquid biopsies in lung cancer, while evaluating the potential biomarkers for early detection, residual disease monitoring, and the treatment response.
Early lung cancer detection
Significant efforts have been made to determine new screening methods for early lung cancer detection, early treatment, and survival improvement [13]. In a high-risk population, the most commonly used lung cancer screening and early detection method is thoracic low-dose CT (LDCT). This method was recommended by the National Lung Screening Trial, which demonstrated the LDCT efficacy for detecting lung cancer, resulting in a 20% reduction in patient mortality when compared to those patients screened using conventional chest x-rays [14,15]. However, there are many LDCT screening limitations, and, as an inefficient diagnostic tool, it has a high false positive rate of 96.6%. These false positives can lead to excessive medical care, unnecessary invasive diagnostic procedures, increased deaths from avoidable surgeries, and psychological overload [16]. In addition, LDCT is associated with recurrent radiation exposure, which can lead to the development of radiation-induced lung cancer [17].
Tumor or cancer biomarkers can be used to detect early stage lung cancer independently or in combination with the LDCT-based screening techniques used for selecting high-risk patients to undergo further diagnostic examinations. They can help increase the proportion of complete surgical resections, which is the most effective curative therapy for local tumors [18]. In order to be as effective as a clinical or early diagnostic device, the biomarker must be present in the bodily fluids, be measurable and reliable, and show a high sensitivity and specificity to the disease being studied [19]. Despite the numerous screening outcomes with successful results, the clinically available lung cancer biomarkers do not have sufficiently high specificities and sensitivities for widespread use [20].
Lung cancer diagnosis
Although a tumor tissue biopsy is the most reliable method for diagnosing cancer in clinical medicine, it is an invasive procedure, it is often unattainable, and it has various limitations in clinical practice [11,21]. A conventional needle biopsy, bronchoscopy, thoracentesis, or other invasive method is required to obtain a sufficient number of samples, depending on the disease site. This usually provides inadequate material, and it represents a single snapshot of the tumor. When the tissue obtained is sufficient for a histopathological analysis, a definitive diagnosis is more likely. However, even if a sufficient biopsy can be obtained, it is an unreliable method in cases of heterogeneous and continuously evolving tumors, because it increases the patient’s risk and discomfort when collecting repetitive samples [21,22].
It is always difficult and sometimes impossible to obtain tumor tissues from patients in order to monitor the tumor dynamics, because most diagnostic procedures are invasive, and the patients dislike repeated approaches. In addition, tumor heterogeneity is subject to selection bias. Moreover, especially for patients suffering from a relapse after receiving chemotherapy or targeted therapy, the original somatic mutation may change [15,23]. Nosaki et al. [24] reported that the rebiopsy rate using an invasive method had overtaken that of the initial biopsy rate (7.8% vs. 1.8%). The median rebiopsy duration was 12.5 days, suggesting that cooperation between the surgical and radiology departments is mandatory. A rebiopsy can expose a patient to a greater risk of complications and delayed results; therefore, the use of noninvasive diagnostic procedures can avoid causing additional distress to the patient [22].
Potentially, a liquid biopsy could be used for a variety of clinical and investigational applications, and lately, the literature has shown that it is a reliable method for the noninvasive evaluation of tumor-specific biomarkers [25]. A liquid biopsy has several advantages, such as its feasibility for collecting multiple specimens at various points before and during treatment, heterogeneous resistance mechanisms, and wider representation of the entire genomic landscape by monitoring tumor genomic changes over time (including the primary tumor and multiple metastatic sites) [11]. When detecting a mutation in a liquid biopsy, one cannot say conclusively that it belongs to the lung mass seen in the imaging. However, although these mutations cannot be distinguished from a second primary metastatic malignancy, they can strengthen the diagnosis of primary lung cancer [26].
There are a variety of biological elements that can be isolated from the peripheral blood, including exosomes, cell-free DNA/circulating tumor DNA (cfDNA/ctDNA), circulating tumor cells (CTCs), and microRNA (miRNA). Additionally, a liquid biopsy also includes tumor-educated platelets (TEPs), tumor-associated antigens (TAA), and tumor-associated autoantibodies (TAAb) [8,12,27,28]. The ctDNA certainly represents the most experiential element of a liquid biopsy in clinical practice with regard to lung cancer patients. Both ctDNA and exosomes carry many characteristics of the tumors from which they arise. They have provided promising results as biomarkers, and they preserve much of the valuable molecular information [29]. However, there are many difficulties associated with this approach, such as the presence of small amounts of secreted tumor components, short half-lives, cell/DNA fragmentation, high tumor cell mutation variation, and tumor source identification failure [30].
a. Exosomes
Exosomes (also called extracellular vesicles) of 40–100 nm in size were first discovered by Johnstone et al. [31] in 1987, and they have been described as microvesicles with 5’-nucleotidase activity. They are produced from multivesicular bodies and released from neoplastic and other cells into the extracellular space and a variety of bodily fluids [32]. Exosomes consist of lipids, proteins, and nucleic acids (DNA, as well as coding and noncoding RNA) from tumor cells, and indicate many tumor characteristics, such as the status of the cells from which they originate and the cellular mechanisms that they engage in [33]. Being relatively stable, they are ideal candidates for liquid biopsy diagnoses [34]. Exosomes play important roles in the proliferation of cancer cells, along with their primary duty in exosome-mediated communication within the tumor environment by transferring biomacromolecules to targeted cells modulating the activities of the recipient cells [34,35].
Exosomes can be selected with the presence of specific protein markers, such as the tetraspanin proteins CD63, CD9, and CD81, and they can also be extracted from body fluids by normal density-gradient centrifugation or ultracentrifugation and visualized by transmission microscopy [12,36]. Rabinowits et al. showed that the circulating tumor exosome levels (exosomal small RNA and exosomal miRNA specific for lung adenocarcinoma) were higher in lung adenocarcinoma patients when compared to a healthy group [37]. Therefore, one might obtain information about a disease’s pathology and prognosis through the exosomal miRNA’s disease-specific gene signature [38].
Exosomal miRNA is enriched in the circulatory system, and it is protected from RNase degradation, when compared to the circulating miRNA. When the exosomes are extracted, the contents (miRNA) can be investigated using different techniques, including the reverse transcription polymerase chain reaction (RT-PCR), sequencing, Western blot, or enzyme-linked immunosorbent assay (ELISA), for somatic mutation, splice variant, gene fusion, and gene or protein expression profiling. As a potential diagnostic biomarker and important mediator of drug resistance, exosomal miRNA has been investigated broadly in lung cancer cases [39].
Jin et al. [40] demonstrated a unique pattern of expression between stage I adenocarcinoma and squamous cell carcinoma (SCC) patients paired with healthy individuals. They used miRNA sequencing to extensively profile tumor-derived exosomal miRNA, and the miRNA profiles showed sensitivities of 80.65% and 83.33% and specificities of 91.67%, and 90.32% for the adenocarcinoma and SCC diagnoses, respectively. Based on these results, one can conclude that for diagnosing NSCLS at an early stage, miRNA is a highly sensitive, noninvasive, and promising biomarker.
Despite the promising results mentioned above, different cell types can release exosomes; therefore, an exosomal miRNA clinical application for an early NSCLC diagnosis is challenging. Other bodily fluids, including pleural effusion, can be used to isolate exosomes in cancer patients [41].
b. cfDNA /ctDNA
Mandel and Metais discovered the presence of circulating cfDNA in the late 1940s [42]. Small fragments of DNA are secreted into the blood circulation from every living cell, and the mechanisms of an increased ctDNA concentration include apoptosis and necrosis, as well as the lysis of viable tumor cells [43]. cfDNA is described as any DNA that is freely circulating in the blood, while ctDNA is the proportion of cfDNA that is specifically released from tumor cells [44]. Because of the ctDNA’s tumor specificity, it can provide a real-time evaluation for specific mutations and diagnostic and prognostic assessments [45,46]. Between malignant and benign diseases, there is a significant difference in the circulating DNA plasma concentrations [47]. Leon et al. [48] discovered that the cfDNA occurred at a much higher level in the blood of cancer patients. After the discovery of ctDNA, a liquid biopsy analysis has become a noninvasive method to diagnose and monitor tumor evolution over time in clinical practice. Cancer patients are of specific interest for exploring the potential of liquid biopsy substitutes for tissue biopsies, because ctDNA detection can mitigate the need for invasive tissue biopsies [12]. A liquid biopsy can also be used to monitor changes during the natural course of the disease or during cancer treatment with the ease of repeated blood sampling [49].
Before its application, there are several challenges, including the major challenge of determining the sensitivity and specificity of ctDNA detection due to its scarcity in the blood when compared to the cfDNA from normal cells [50]. Some studies demonstrated that the quantification and analysis of the cfDNA in the blood during the early disease stages was challenging due to the very low concentration (approximately 0.01%–1%) of the ctDNA fraction [51]. The traditional ctDNA analytic approaches can be broadly divided into amplification and sequencing-based methods. Moreover, highly sensitive blood-based assessments have been developed and used extensively to test the cfDNA at very low concentrations in order to detect ctDNA point mutations. These consist of polymerase chain reaction (PCR)-based techniques [digital PCR, droplet digital PCR, real-time quantitative PCR (qPCR), and peptide nucleic acid clamp-based PCR], beads, emulsions, amplification, and magnetics (BEAMing), pyrophosphorolysis-activated polymerization, and next-generation sequencing technologies. Unfortunately, the methods based on DNA amplification cannot be applied as routine diagnostic tools, because they are time-consuming, expensive, and require large-volume samples (> 5 ml) [51,52]. Hu et al. [53] reported the use of nanomaterials as biosensors in patient serum samples for ultrasensitive ctDNA detection. With this strategy, the treatment responses and patient monitoring both during and after treatment have shown promising results.
Other studies have demonstrated elevated ctDNA levels in cancer patients, as well as the correlation of these levels with the tumor disease burden, tumor response, and survival outcome [54,55]. One study about cfDNA quantification showed that the plasma DNA concentration, as determined by the qPCR of the human telomerase reverse transcriptase gene, was almost eight-times higher in the patients with lung cancer than in the patients with cigarette smoking histories and those at high risk for lung cancer development. Therefore, for patients with a higher risk, this assay is an extremely sensitive and specific noninvasive approach [55]. Newman et al. [56] also determined that the cfDNA levels were significantly correlated with the tumor volume, and that it is possible to detect disease progression earlier when using them than when using radiological approaches. However, if the structure of the tumor tissue and the expected mutations are not known in advance, the role of cfDNA genotype scanning for somatic mutations remains unknown.
c. Methylation
Some studies have reported that tissue-specific DNA can be identified using the methylation information of the circulating DNA. Methylation-based epigenetic markers are promising for the early detection of lung cancer [57,58]. DNA methylation is necessary for a living cell to function, and it is a naturally occurring process. However, because DNA methylation can also occur in the normal tissue surrounding the tumor, the specificity of methylated DNA as a biomarker may be low [11].
Hypermethylation has been shown in cancer patients, and it functions via gene alteration regulation to promote carcinogenesis [59]. Methylated DNA is remarkably stable, it generally exists during the early stages of carcinogenesis, and it can be found in different bodily fluids [59,60]. Even though the epigenetic changes are not unique for any tumor, the methylation of promoter regions within the genomic cytosine-guanine islands leads to gene expression regulation and gene silencing [61]. Moreover, some tumor suppressor genes are frequently methylated and downregulated in specific tumors, which could be useful for determining the diagnosis and prognosis [61]. In 2010, Bailey et al. [62] revealed an efficient and reproducible method for detecting methylation markers from small quantities of DNA in the circulating blood.
d. CTCs
Ashworth described the presence of CTCs in the bloodstream of cancer patients for the first time in 1869 [63]. CTCs are detached from primary tumor sites and/or metastatic sites to circulate in the bloodstream, where they can be found as either single cells or clusters, and they contribute to cancer progression and metastasis development. CTCs are closely associated with the tumor size, progression, metastasis, relapse, and prognosis [64,65]. Some previous studies have suggested that CTCs could be used as biomarkers for determining the prognosis and predicting and monitoring the treatment response [65,66]. When compared to ctDNA, a CTC analysis provides the opportunity to study whole cells that contain DNA, RNA, and protein-based molecular profiling [67].
Thus far, the only US Food and Drug Administration approved CTC detection system for prognostic purposes in metastatic breast, colorectal, or prostate cancer is CellSearch (Veridex, LLC., Raritan, NJ, USA) [68,69]. In early diagnosis, CTCs can be missed because of their low concentration in the blood (1–10 CTCs per 10 ml), epithelial to mesenchymal transition, and downregulation of epithelial markers during progression [70]. Another antibody-mediated capture technology, the CTC-chip, has detected CTCs in metastatic tumors, including lung cancer, at a rate of almost 100%, and in the peripheral blood of patients with solid tumors with approximately 50% purity [69,71]. In lung cancer, different techniques have been used to detect CTCs and evaluate their roles; however, the clinical application of CTCs as predictive and prognostic biomarkers is still under investigation. Moreover, the value of CTCs in NSCLC patients has not yet been determined due to the lack of standardization for the current methods [72].
e. miRNA
miRNA is a small noncoding RNA gene product that ranges between 19 and 22 base pairs, which regulates gene expression by binding to specific targeted messenger RNA (mRNA) sequences. It plays a key role in modulating various cellular processes, including proliferation, cell migration, and apoptosis, and it has also been implicated in several pathological phenotypes. The most common cell-free RNA (cfRNA) molecule in the blood is miRNA [12,73,74], which can be carried in exosomes, apoptotic bodies, protein-miRNA complexes, and TEPs [12]. miRNA serves as a tumor suppressor and oncogene in cancer cells [75].
Different cancer types are associated with different miRNA expression patterns, and the structure of the miRNA in the circulating blood is similar to that of a primary solid tumor [76]. Indeed, the let-7 family is a novel biomarker for lung cancer [77]. In one study evaluating miRNA as a potential biomarker for NSCLC, the diagnostic value of a plasma miRNA set, including miRNA-21, miRNA-126, miRNA-210, and miRNA-486-5p, revealed 86.2% sensitivity and 96.55% specificity. Additionally, the study reported that altered expressions were previously confirmed in lung tumor tissues [78]. miRNA is highly stable in the blood, and thus, it may become an ideal biomarker for lung cancer detection [79].
f. Other biomarkers
Many other biomarkers can be obtained from liquid biopsy samples, and these could be used for the early detection and screening of lung cancer. During the early stages of lung cancer, TAAs, such as the carcinoembryonic antigen, carbohydrate antigen (CA) 125, CA199, neuron specific enolase, and cytokeratin 19 fragment 21-1, are rarely released into the bloodstream. Because of their poor sensitivities and specificities, they cannot detect the various heterogeneous lung cancer types [80]. However, TAAbs, which are autoantibodies against overexpressed, mutated, misfolded, or aberrant autologous cellular antigens, are very stable, and they can be detected at high levels during the early stages of lung cancer [81]. Sullivan et al. [82] conducted the largest lung cancer screening program with 12,000 participants in Scotland. It consisted of a randomized controlled trial of patients at high risk of developing lung cancer, and it was run based on a 7-AAb panel.
Hemostasis is the main role of the platelets, but they may be a good platform for detecting rearrangements and for diagnosis, because, apart from hemostasis, they play outstanding roles in the systemic and local responses to tumor growth [83,84]. Platelets take up the tumor-related RNA released from tumor cells via several microvesicle-dependent or independent mechanisms, resulting in TEPs [85]. In addition, platelets pass through specific pre-mRNA assembly in response to the activation of the platelet surface receptors, leading to a unique mRNA profile that could possibly be used for a cancer diagnosis [86].
Postsurgical and treatment response monitoring
The standard method for analyzing the genetic changes in cancer tissue is a repeated tumor biopsy. However, this is an invasive and impractical technique that often results in an insufficient tissue sample [87].
Recurrence and metastases may develop in approximately 30%–50% of NSCLC patients [88]. After surgery, routine biomarker monitoring using a liquid biopsy could reveal recurrence 1 to 2 years earlier than a radiographic screening, as well as reveal those who would benefit from certain therapy types (i.e. chemotherapy and radiotherapy) [89]. A liquid biopsy can be used to determine somatic mutations before treatment, and dynamically during treatment, enabling the quantification of the minimal residual disease and emergence of therapy resistance, as well as monitoring the therapy response. Recently, many studies have focused on dynamic CTCs/ctDNA monitoring to predict a relapse as soon as possible. The results have shown that the ctDNA, with a higher sensitivity, was more favorable and reliable than the CTCs [11,12,90].
Although the lung cancer survival rate is low, molecularly targeted therapies for gene alterations, such as the epidermal growth factor receptor (EGFR), anaplastic lymphoma kinase (ALK), and ROS1, have radically changed the treatment approach and prognosis of the disease [91,92]. However, the long-term efficacy of these agents is limited by tumor heterogeneity, leading to multiple resistance mechanisms being developed during targeted therapy [92,93]. In Turkey, gene sequence analyses for ALK, KRAS, BRAF, ROS1, EGFR, and MET using cfDNA are being conducted at the medical genetics departments of certain universities.
Lung cancer treatment has dramatically evolved over the last decade, mainly because of the clinical use of targeted and personalized therapies. Since lung cancer is a highly heterogeneous disease, certain patients do not respond to a targeted agent while having an activated oncogene, and some are faced with rapid disease progression, although they showed an initial objective response [94].
In clinical practice, the plasma genotyping for EGFR sensitizing or T790M-mutations through cfDNA extraction can predict the response to EGFR and EGFR-tyrosine kinase inhibitor (EGFR-TKI) therapy, and it can monitor the resistance possibilities [95]. Approximately 50%–60% of the patients with EGFR mutations in exon 20 (T790M) exhibit progression while undergoing treatment with EGFR-TKIs due to therapy resistance [96].
Tumors can resist autoimmune antitumor activity by modulating immune checkpoint inhibitors and immune checkpoint proteins, like programmed death ligand 1 (PD-L1) and programmed cell death 1 (PD-1) [97]. The expression of PD-L1 is elevated in many types of cancer, and it often goes along with the tumor mutational burden, suggesting a poor prognosis and predicting the response to targeted therapy drugs [98,99]. A tissue biopsy shows only a snapshot of the PD-L1 expression, while a liquid biopsy has the ability to dynamically examine the whole molecular picture of the tumor tissue by using CTCs as a substrate for the analysis, because PD-L1 is a dynamic biomarker that can be induced by targeted therapy, chemotherapy, or radiation therapy [100]. Immunotherapy has become an effective approach for the treatment of NSCLC, and regulatory agencies in both the US and EU have already approved PD-L1/PD-1 immunotherapy [101].
Fusion genes, which encode fusion proteins, generate noncoding RNA, and contribute to tumor progression, are caused by aberrant chromosomal translocations [102]. NSCLC cases exhibit the echinoderm microtubule-associated protein-like 4 (EML4)-ALK fusion gene at a rate of approximately 4%–5%. EML4-ALK translocation activates ALK-TKI and generates tumor proliferation, migration, and invasion [103]. Veldore et al. [104] recommended that targeted therapy could be used for 8%–10% of all the EGFR mutation-negative NSCLC patients who have translocations in EML4-ALK, ROS1-ALK, and KIP4-ALK.
Overall, it has become mandatory that universal experimental and therapeutic research regarding advanced molecular diagnostics be done to solve common resistance mechanisms with the development of next-generation agents.
As a conclusion, although a tissue biopsy is still the accepted standard for diagnosing lung cancer, a liquid biopsy can offer an alternative or supportive technique when a tissue biopsy is insufficient or unobtainable. The development of analytical technologies and the knowledge gained from large-scale sequencing projects have provided possibilities for individualized diagnoses and targeted drug treatments. Combining a liquid biopsy with a tumor biopsy could introduce additional qualifications toward improvements in NSCLC patient management. With the growing interest in molecular testing in every field of cancer, liquid biopsies have shown considerable promise for compiling precious information about cancer genetic alterations in almost real time. We strongly believe that there is an urgent need for biomarkers with high accuracy, which might improve early stage lung cancer detection, predict the treatment response, and anticipate tumor relapses that are not yet visible on imaging. In addition, they can provide a rapid and dynamic assessment of progressive resistance mechanisms.
Declaration of conflicting interests
The authors declared no conflicts of interest with respect to the authorship and/or publication of this article.
Funding
The authors received no financial support.
Reference
1) Siegel RL, Miller KD, Jemal A. Cancer statistics, 2017. CA Cancer J Clin 2017; 67: 177-193
2) Woodard GA, Jones KD, Jablons DM. Lung cancer staging and prognosis. Cancer Treat Res 2016; 170: 47-75.
3) Howlader N, Noone AM, Krapcho M, Miller D, Bishop K, Kosary CL, et al. SEER cancer statistics review, 1975-2014. Bethesda, MD: National Cancer Institute; 2017.
4) Kris MG, Gaspar LE, Chaft JE, Kennedy EB, Azzoli CG, Ellis PM, et al. Adjuvant systemic therapy and adjuvant radiation therapy for stage I to IIIA completely resected non–small-cell lung cancers: American Society of Clinical Oncology/Cancer Care Ontario clinical practice guideline update. J Clin Oncol 2017; 35: 2960-74.
5) Chansky K, Detterbeck FC, Nicholson AG, Rusch VW, Vallières E, Groome P, et al. The IASLC lung cancer staging project: external validation of the revision of the TNM stage groupings in the eighth edition of the TNM classification of lung cancer. J Thorac Oncol 2017; 12: 1109-21.
6) Ettinger DS, Wood DE, Aisner DL, Akerley W, Bauman J, Chirieac LR, et al. Non-small cell lung cancer, version 5.2017, NCCN clinical practice guidelines in oncology. J Natl Compr Canc Netw 2017; 15: 504-35.
7) Früh M, Rolland E, Pignon JP, Seymour L, Ding K, Tribodet H, et al. Pooled analysis of the effect of age on adjuvant cisplatin-based chemotherapy for completely resected non-small-cell lung cancer. J Clin Oncol 2008; 26: 3573-81.
8) Nguyen DX, Bos PD, Massague J. Metastasis: from dissemination to organ-specific colonization. Nat Rev Cancer 2009; 9: 274-84.
9) Siegel RL, Miller KD, Jemal A. Cancer statistics, 2016. CA Cancer J Clin 2016; 66: 7-30.
10) Eichmüller SB, Osen W, Mandelboim O, Seliger B. Immune, modulatory microRNAs involved in tumor attack and tumor immune escape. J Natl Cancer Inst 2017; 109(10). doi: 10.1093/jnci/djx034.
11) Santarpia M, Karachaliou N, González-Cao M, et al. Feasibility of cell-free circulating tumor DNA testing for lung cancer. Biomark Med 2016; 10: 417-30.
12) Tarro G, Perna A, Esposito C. Early diagnosis of lung cancer by detection of tumor liberated protein. J Cell Physiol 2005; 203: 1-5.
13) Smith RA, Cokkinides V, Brooks D, Saslow D, Shah M, Brawley OW. Cancer screening in the United States, 2011. CA Cancer J Clin 2011; 61: 8-30.
14) Ostrowski M, Marjanski T, Rzyman W. Low-dose computed tomography screening reduces lung cancer mortality. Adv Med Sci 2018; 63: 230-6.
15) National Lung Screening Trial Research Team. The national lung screening trial: overview and study design. Radiology 2011; 258: 243-53.
16) Croswell JM, Baker SG, Marcus PM, Clapp JD, Kramer BS. Cumulative incidence of false-positive test results in lung cancer screening: a randomized trial. Ann Intern Med 2010; 152: 505-12, W176-80.
17) Rampinelli C, De Marco P, Origgi D, Maisonneuve P, Casiraghi M, Veronesi G, et al. Exposure to low dose computed tomography for lung cancer screening and risk of cancer: secondary analysis of trial data and risk-benefit analysis. BMJ 2017; 356: j347.
18) Lackey A, Donington JS. Surgical management of lung cancer. Semin Intervent Radiol 2013; 30: 133-40.
19) Mayeux R. Biomarkers: potential uses and limitations. NeuroRx 2004; 1: 182-8.
20) Zamay TN, Zamay GS, Kolovskaya OS, Zukov RA, Petrova MM, Gargaun A, et al. Current and prospective protein biomarkers of lung cancer. Cancers (Basel) 2017; 9: 155.
21) Murtaza M, Dawson SJ, Tsui DW, Gale D, Forshew T, Piskorz AM, et al. Non-invasive analysis of acquired resistance to cancer therapy by sequencing of plasma DNA. Nature 2013; 497: 108.
22) Poroyko V, Mirzapoiazova T, Nam A, Mambetsariev I, Mambetsariev B, Wu X, et al. Exosomal miRNAs species in the blood of small cell and non-small cell lung cancer patients. Oncotarget 2018; 9: 19793.
23) Ilié M, Hofman P. Pros: can tissue biopsy be replaced by liquid biopsy? Transl Lung Cancer Res 2016; 5: 420-3.
24) Nosaki K, Satouchi M, Kurata T, Yoshida T, Okamoto I, Katakami N, et al. Rebiopsy status among non-small cell lung cancer patients in Japan: a retrospective study. Lung Cancer 2016; 101: 1-8.
25) Siravegna G, Marsoni S, Siena S, Bardelli A. Integrating liquid biopsies into the management of cancer. Nat Rev Clin Oncol 2017; 14: 531-48.
26) Pu Q, Huang Y, Lu Y, Peng Y, Zhang J, Feng G, et al. Tissue specific and plasma microRNA profiles could be promising biomarkers of histological classification and TNM stage in non-small cell lung cancer. Thorac Cancer 2016; 7: 348-54.
27) Chapman CJ, Murray A, McElveen JE, Sahin U, Luxemburger U, Türeci, et al. Autoantibodies in lung cancer: possibilities for early detection and subsequent cure. Thorax 2008; 63: 228-33.
28) Raez LE, Manca P, Rolfo C, Singh V. ROS-1 Rearrangements in Circulating Tumor Cells. J Thorac Oncol 2018; 13: e71-2.
29) Pantel K, Alix-Panabières C. Real-time liquid biopsy in cancer patients: fact or fiction? Cancer Res 2013; 73: 6384-8.
30) Hiley CT, Le Quesne J, Santis G, Sharpe R, de Castro DG, Middleton G, et al. Challenges in molecular testing in non-small-cell lung cancer patients with advanced disease. Lancet 2016; 388: 1002-11.
31) Johnstone RM, Adam M, Hammond JR, Orr L, Turbide C. Vesicle formation during reticulocyte maturation. Association of plasma membrane activities with released vesicles (exosomes). J Biol Chem 1987; 262: 9412-20.
32) Raposo G, Stoorvogel W. Extracellular vesicles: exosomes, microvesicles, and friends. J Cell Biol 2013; 200: 373-83.
33) Zhou L, Lv T, Zhang Q, Zhu Q, Zhan P, Zhu S, et al. The biology, function and clinical implications of exosomes in lung cancer. Cancer Lett 2017; 407: 84-92.
34) Meehan K, Vella LJ. The contribution of tumour-derived exosomes to the hallmarks of cancer. Crit Rev Clin Lab Sci 2016; 53: 121-31.
35) Weidle UH, Birzele F, Kollmorgen G, Rueger R. The multiple roles of exosomes in metastasis. Cancer Genomics Proteomics 2017; 14: 1-15.
36) Rani S, O’Brien K, Kelleher FC, Corcoran C, Germano S, Radomski MW, et al. Isolation of exosomes for subsequent mRNA, microRNA, and protein profiling. Methods Mol Biol 2011; 784: 181-95.
37) Rabinowits G, Gercel-Taylor C, Day JM, Taylor DD, Kloecker GH. Exosomal microRNA: a diagnostic marker for lung cancer. Clin Lung Cancer 2009; 10: 42-6.
38) Takahashi RU, Prieto-Vila M, Hironaka A, Ochiya T. The role of extracellular vesicle microRNAs in cancer biology. Clin Chem Lab Med 2017; 55: 648-56.
39) Reclusa P, Taverna S, Pucci M, Durendez E, Calabuig S, Manca P, et al. Exosomes as diagnostic and predictive biomarkers in lung cancer. J Thorac Dis 2017; 9: S1373-82.
40) Jin X, Chen Y, Chen H, Fei S, Chen D, Cai X, et al. Evaluation of tumor-derived exosomal miRNA as potential diagnostic biomarkers for early-stage non-small cell lung cancer using next-generation sequencing. Clin Cancer Res 2017; 23: 5311-9.
41) Han HS, Yun J, Lim SN, Han JH, Lee KH, Kim ST, et al. Downregulation of cell-free miR-198 as a diagnostic biomarker for lung adenocarcinoma-associated malignant pleural effusion. Int J Cancer 2013; 133: 645-52.
42) Mandel P, Metais P. Nucleic acids of blood plasma in humans. C R Seances Soc Biol Fil 1948; 142: 241-3.
43) Thierry AR, El Messaoudi S, Gahan PB, Anker P, Stroun M. Origins, structures, and functions of circulating DNA in oncology. Cancer Metastasis Rev 2016; 35: 347-76.
44) Schwarzenbach H, Hoon DS, Pantel K. Cell-free nucleic acids as biomarkers in cancer patients. Nat Rev Cancer 2011; 11: 426-37.
45) Hashad D, Sorour A, Ghazal A, Talaat I. Free circulating tumor DNA as a diagnostic marker for breast cancer. J Clin Lab Anal 2012; 26: 467-72.
46) Salvianti F, Pinzani P, Verderio P, Ciniselli CM, Massi D, De Giorgi V, et al. Multiparametric analysis of cell-free DNA in melanoma patients. PLoS One 2012; 7: e49843.
47) Zaher ER, Anwar MM, Kohail HM, El-Zoghby SM, Abo-El-Eneen MS. Cell-free DNA concentration and integrity as a screening tool for cancer. Indian J Cancer 2013; 50: 175-83.
48) Leon SA, Shapiro B, Sklaroff DM, Yaros MJ. Free DNA in the serum of cancer patients and the effect of therapy. Cancer Res 1977; 37: 646-50.
49) Vendrell JA, Mau-Them FT, Béganton B, Godreuil S, Coopman P, Solassol J. Circulating cell free tumor DNA detection as a routine tool for lung cancer patient management. Int J Mol Sci 2017; 18: 264.
50) Ma M, Zhu H, Zhang C, Sun X, Gao X, Chen G. “Liquid biopsy”-ctDNA detection with great potential and challenges. Ann Transl Med 2015; 3: 235.
51) Mayo-de-Las-Casas C, Garzon Ibanez M, Jordana-Ariza N, García-Peláez B, Balada-Bel A, Villatoro S, et al. An update on liquid biopsy analysis for diagnostic and monitoring applications in non-small cell lung cancer. Expert Rev Mol Diagn 2018; 18: 35-45.
52) García-Olmo DC, Domínguez C, García-Arranz M, Anker P, Stroun M, García-Verdugo JM, et al. Cell-free nucleic acids circulating in the plasma of colorectal cancer patients induce the oncogenic transformation of susceptible cultured cells. Cancer Res 2010; 70: 560.
53) Hu P, Zhang S, Wu T, Ni D, Fan W, Zhu Y, et al. Fe–Au nanoparticle coupling for ultrasensitive detections of circulating tumor DNA. Advanced Materials 2018; 1801690.
54) Brevet M, Johnson ML, Azzoli CG, Ladanyi M. Detection of EGFR mutations in plasma DNA from lung cancer patients by mass spectrometry genotyping is predictive of tumor EGFR status and response to EGFR inhibitors. Lung Cancer 2011; 73: 96-102.
55) Sozzi G, Conte D, Leon M, Cirincione R, Roz L, Ratcliffe C, et al. Quantification of free circulating DNA as a diagnostic marker in lung cancer. J Clin Oncol 2003; 21: 3902-8.
56) Newman AM, Bratman SV, To J, Wynne JF, Eclov NC, Modlin LA, et al. An ultrasensitive method for quantitating circulating tumor DNA with broad patient coverage. Nat Med 2014; 20: 548-54.
57) Lehmann-Werman R, Neiman D, Zemmour H, Moss J, Magenheim J, Vaknin-Dembinsky A, et al. Identification of tissue-specific cell death using methylation patterns of circulating DNA. Proc Natl Acad Sci USA 2016; 113: E1826-34.
58) Lerner L, Winn R, Hulbert A. Lung cancer early detection and health disparities: the intersection of epigenetics and ethnicity. J Thorac Dis 2018; 10: 2498-507.
59) Esteller M, Herman JG. Cancer as an epigenetic disease: DNA methylation and chromatin alterations in human tumours. J Pathol 2002; 196: 1-7.
60) Heyn H, Esteller M. DNA methylation profiling in the clinic: applications and challenges. Nat Rev Genet 2012; 13: 679-92.
61) Shivapurkar N, Gazdar AF. DNA methylation based biomarkers in non-invasive cancer screening. Curr Mol Med 2010; 10: 123-32.
62) Bailey VJ, Zhang Y, Keeley BP, Yin C, Pelosky KL, Brock M, et al. Single-tube analysis of DNA methylation with silica superparamagnetic beads. Clin Chem 2010; 56: 1022-5.
63) Ashworth TR. A case of cancer in which cells similar to those in the tumors were seen in the blood after death. Aust Med J 1869; 14: 146-9.
64) Plaks V, Koopman CD, Werb Z. Cancer. Circulating tumor cells. Science 2013; 341: 1186-8.
65) Krebs MG, Metcalf RL, Carter L, Brady G, Blackhall FH, Dive C. Molecular analysis of circulating tumour cells—biology and biomarkers. Nat Rev Clin Oncol 2014; 11: 129-44.
66) Alix-Panabières C, Pantel K. Circulating tumor cells: liquid biopsy of cancer. Clin Chem 2013; 59: 110-8.
67) Calabuig-Fariñas S, Jantus-Lewintre E, Herreros-Pomares A, Camps C. Circulating tumor cells versus circulating tumor DNA in lung cancer-which one will win? Transl Lung Cancer Res 2016; 5: 466-82.
68) Cohen SJ, Punt CJ, Iannotti N, Saidman BH, Sabbath KD, Gabrail NY, et al. Relationship of circulating tumor cells to tumor response, progression-free survival, and overall survival in patients with metastatic colorectal cancer. J Clin Oncol 2008; 26: 3213-21.
69) Krebs MG, Sloane R, Priest L, Lancashire L, Hou JM, Greystoke A, et al. Evaluation and prognostic significance of circulating tumor cells in patients with non-small-cell lung cancer. J Clin Oncol 2011; 29: 1556-63.
70) Allard WJ, Matera J, Miller MC, Repollet M, Connelly MC, Rao C, et al. Tumor cells circulate in the peripheral blood of all major carcinomas but not in healthy subjects or patients with nonmalignant diseases. Clin Cancer Res 2004; 10: 6897-904.
71) Nagrath S, Sequist LV, Maheswaran S, Bell DW, Irimia D, Ulkus L, et al. Isolation of rare circulating tumour cells in cancer patients by microchip technology. Nature 2007; 450: 1235-9.
72) Hofman V, Bonnetaud C, Ilie MI, Vielh P, Vignaud JM, Flejou JF, et al. Preoperative circulating tumor cell detection using the isolation by size of epithelial tumor cell method for patients with lung cancer is a new prognostic biomarker. Clin Cancer Res 2011; 17: 827-35.
73) Iorio MV, Croce CM. MicroRNAs in cancer: small molecules with a huge impact. J Clin Oncol 2009; 27: 5848-56.
74) Ambros V. MicroRNA pathways in flies and worms. Cell 2003; 113: 673-6.
75) Manikandan J, Aarthi JJ, Kumar SD, Pushparaj PN. Oncomirs: the potential role of non-coding microRNAs in understanding cancer. Bioinformation 2008; 2: 330-4.
76) Chen X, Ba Y, Ma L, Cai X, Yin Y, Wang K, et al. Characterization of microRNAs in serum: a novel class of biomarkers for diagnosis of cancer and other diseases. Cell Res 2008; 18: 997-1006.
77) Xie P, Li X, Tan X, Sun X, Wang C, Yu J. Sequential serum let-7 is a novel biomarker to predict accelerated reproliferation during fractional radiotherapy in lung cancer. Clin Lung Cancer 2016; 17: e95-101.
78) Shen J, Todd NW, Zhang H, Yu L, Lingxiao X, Mei Y, et al. Plasma microRNAs as potential biomarkers for non-small-cell lung cancer. Lab Invest 2011; 91: 579-87.
79) Mitchell PS, Parkin RK, Kroh EM, Fritz BR, Wyman SK, Pogosova-Agadjanyan EL, et al. Circulating microRNAs as stable blood-based markers for cancer detection. Proc Natl Acad Sci USA 2008; 105: 10513-8.
80) Murray A, Chapman CJ, Healey G, Peek LJ, Parsons G, Baldwin D, et al. Technical validation of an autoantibody test for lung cancer. Ann Oncol 2010; 21: 1687-93.
81) Jett JR, Peek LJ, Fredericks L, Jewell W, Pingleton WW, Robertson JF. Audit of the autoantibody test, early CDT(R)-lung, in 1600 patients: an evaluation of its performance in routine clinical practice. Lung Cancer 2014; 83: 51-5.
82) Sullivan F, Dorward A, Mair F, Schembri S, Vedhara K, Kendrick D, et al. P2.06-038 an RCT of the detection of autoantibodies to tumor antigens in lung cancer using the early CDT-lung test in Scotland (ECLS) in 12208 Study Subjects. J Thorac Oncol 2017; 12: S1095.
83) Leslie M. Cell biology. Beyond clotting: the powers of platelets. Science 2010; 328: 562-4.
84) Best MG, Sol N, Kooi I, Tannous J, Westerman BA, Rustenburg F, et al. RNA seq of tumor educated platelets enables blood based pan cancer, multiclass, and molecular pathway cancer diagnostics. Cancer Cell 2015; 28: 666 76.
85) Pérez-Callejo D, Romero A, Provencio M, Torrente M. Liquid biopsy based biomarkers in non-small cell lung cancer for diagnosis and treatment monitoring. Transl Lung Cancer Res 2016; 5: 455‑65.
86) Nilsson RJ, Balaj L, Hulleman E, van Rijn S, Pegtel DM, Walraven M, et al. Blood platelets contain tumor-derived RNA biomarkers. Blood 2011; 118: 3680-3.
87) Yung TK, Chan KA, Mok TS, Tong J, To KF, Lo YD. Single-molecule detection of epidermal growth factor receptor mutations in plasma by microfluidics digital PCR in non–small cell lung cancer patients. Clin Cancer Res 2009; 15: 2076-84.
88) Uramoto H, Tanaka F. Recurrence after surgery in patients with NSCLC. Transl Lung Cancer Res. AME Publications 2014; 3: 242-9.
89) Chudasama DY, Aladag Z, Felicien MI, Hall M, Beeson J, Asadi N, et al. Prognostic value of the DNA integrity index in patients with malignant lung tumors. Oncotarget 2018; 9: 21281.
90) Chae YK, Davis AA, Carneiro BA, Chandra S, Mohindra N, Kalyan A, et al. Concordance between genomic alterations assessed by next-generation sequencing in tumor tissue or circulating cell-free DNA. Oncotarget 2016; 7: 65364-73.
91) Hanna N, Johnson D, Temin S, Baker Jr S, Brahmer J, Ellis PM, et al. Systemic therapy for stage IV non-small-cell lung cancer: American Society of Clinical Oncology clinical practice guideline update. J Clin Oncol 2017; 35: 3484-515.
92) Karachaliou N, Sosa AE, Barron FB, Gonzalez Cao M, Santarpia M, Rosell R. Pharmacological management of relapsed/refractory NSCLC with chemical drugs. Expert Opin Pharmacother 2017; 18: 295-304.
93) Tsui DWY, Murtaza M, Wong ASC, Rueda OM, Smith CG, Chandrananda D, et al. Dynamics of multiple resistance mechanisms in plasma DNA during EGFR targeted therapies in non-small cell lung cancer. EMBO Molecular Medicine 2018; 10: e7945.
94) Santarpia M, Funel N, Ali A, Giovannetti E. Liquid biopsies to optimize therapeutic efficacy in unresponsive lung cancer patients. Expert Opinion on Drug Metabolism & Toxicology 2018. doi: 10.1080/17425255.2018.1491965
95) Liang H, Huang J, Wang B, Liu Z, He J, Liang W. The role of liquid biopsy in predicting post-operative recurrence of non-small cell lung cancer. J Thorac Dis 2018; 10: S838-45.
96) Ohashi K, Maruvka YE, Michor F, Pao W. Epidermal growth factor receptor tyrosine kinase inhibitor-resistant disease. J Clin Oncol 2013; 31: 1070-80.
97) Herbst RS, Soria J, Kowanetz M, Fine GD, Hamid O, Gordon MS, et al. Predictive correlates of response to the anti-PD-L1 antibody MPDL3280A in cancer patients. Nature 2014; 515: 563.
98) Rizvi NA, Hellmann MD, Snyder A, Kvistborg P, Makarov V, Havel JJ, et al. Cancer immunology. Mutational landscape determines sensitivity to PD-1 blockade in non-small cell lung cancer. Science 2015; 348: 124-8.
99) Khunger M, Hernandez AV, Pasupuleti V, Rakshit S, Pennell NA, Stevenson J, et al. Programmed cell death 1 (PD-1) ligand (PD-L1) expression in solid tumors as a predictive biomarker of benefit from PD-1/PD-L1 axis inhibitors: a systematic review and meta-analysis. JCO Precis Oncol 2017; 1: 1-15.
100) Ilié M, Szafer-Glusman E, Hofman V, et al. Detection of PD-L1 in circulating tumor cells and white blood cells from patients with advanced non-small cell lung cancer. Ann Oncol 2017; 29: 193-9, 923-7534.
101) Reck M, Rodríguez-Abreu D, Robinson AG, Hui R, Csőszi T, Fülöp A, et al. Pembrolizumab versus chemotherapy for PD-L1-positive non-small-cell lung cancer. N Engl J Med 2016; 375: 1823-33.
102) Tan S, Gou Q, Pu W, Guo C, Yang Y, Wu K, et al. Circular RNA F-circEA produced from EML4-ALK fusion gene as a novel liquid biopsy biomarker for non-small cell lung cancer. Cell Res 2018; 28: 693-5.