
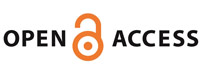
Summary
Background: The robotic assisted thoracoscopic surgery (RATS) spreads rapidly across the whole world. In this paper, the objective was to present the training experience on RATS in our clinic along with the literature.Materials and Methods: Sixty-three consecutive patients, who had undergone RATS between February 2014 and December 2018, were included in this retrospective non-randomized study. The training period was designed as three phases. Twelve patients with solid and cystic mass lesions in the mediastinum and chest wall were included in group Phase 1. The group Phase 2 consisted of 16 patients who had thymoma and benign pulmonary mass lesions, and 35 consecutive patients were enrolled into group Phase 3 group who had undergone lobectomy.
Results: The patients in all groups were compared in respect of the preoperative, perioperative and postoperative results. The docking time was significantly shorter in Phase 3 patients compared to the groups Phase 1 and 2 (p < 0.05). In the lobectomy group (Phase 3), as the console time and operation time was significantly shorter after the 20th patient than the prior patients, first 20 patients were accepted as the learning curve (p < 0.05). In the lobectomy group, in three patients the operation was converted to open surgery during the perioperative period (4.7%). No mortality was observed during the 30-day follow-up. One patient died in the 6th month of the long-term follow-up. The mean follow-up duration was 28.4 months.
Conclusions: RATS is a safe, feasible, and minimally invasive method, which has low complication rates with a satisfying and short learning curve.
Introduction
The robotic surgery spread rapidly across the world following its introduction in the early 2000s. The implementation of the minimally invasive surgery with high technology got an edge over the open surgery depending on the surgical tools with the maneuver capacity of a human wrist, filtration of the physiological tremor (6-Hz motion filter), good dissection capacity in hard-to-access fields, 3D imaging possibility, and 10x magnification capacity [1]. However, the control of the surgical tools with the joystick on the console (instead of directly next to the patient), the absence of tactual sense and position impingement of the robotic arms are its disadvantages. These advantages can only be eliminated with a well-thought-out training. It was reported that the complications, which might be related to surgeons, who used to perform open surgery or video-assisted thoracoscopic surgery (VATS), might have high mortality rates [2]. Therefore, the robotic surgery training is essential. The developments in robotic technology enabled the introduction of different robotic arms for different specialties, which made robotic surgery easier. Thus, different robotic trainings are necessary for each surgical specialty.As there was no training clinic in the clinic of thoracic surgery, the objective was to evaluate the RATS training process extending from simple interventions to lobectomies in the light of the relevant literature.
Methods
Sixty-three patients, who had undergone RATS between November 2014 and December 2018, were selected from a total of 605 patients who had undergone a surgical intervention, and included in this retrospective study. Twelve patients, who did not need vascular and/or bronchial resection and ligation before robotic surgery and their operation fields were close to these structures were included in group Phase 1. 16 patients, who had benign and malign lesions in the close neighborhood to the vascular, bronchial structures needing ligation/closure of the vascular and minor bronchial structures, were gathered in group Phase 2. After the completion of this phase, 35 patients, who had undergone lobectomy, were included in group Phase 3.All patients had undergone preoperative chest computed tomography examination. Patients, who were diagnosed with malignancy or were considered to have a malignancy, were examined with PET/CT and cranial MRI. Standard blood count, biochemical analysis, and pulmonary function testing were also performed. Patients, who were scheduled for RATS but had FEV1 < 1.5 L and had central lesions or lesions larger than 5.1 cm, were not included in the RATS lobectomy program.
All interventions were done by a single surgeon and in same operating room with the da Vinci Robotic System SI (Intuitive Surgical Inc., Mountain View, California, USA).
The operation time for each patient was recorded as the sum of docking time, console time and closure duration. The docking time was defined as the time between the first incision and the sitting of the surgeon at the console. The console time was defined as the time starting from the sitting of the surgeon at the console and ending with the removal of the resected material and undocking of the robotic arms from the patient following the bleeding and air leak control. The closure time started from the undocking of the robot and ended with the closure of the last incision.
Only one thoracic drain was inserted in all resections (28F thoracic tube, thorax drainage system, Bıcakcılar, Istanbul, Turkey). The drains were removed after the daily drained volume declined below 200 mL and no air leak was determined.
Surgical Technique
Following the double-lumen intubation, the patient was positioned in the semi-lateral position in thymoma cases and in the lateral decubitus position in other cases. The ports were so positioned that they were as far as possible to the lesion in order to enable the robotic arms to function smoothly. The distance between the ports was approximately 8-10 cm. The robot was directly positioned at the front of the lesion. Docking was adjusted so that the arm might move towards the lesion and the robot and the transverse axis of cart angle to the vertebral column was 30-45°. The surgeon next the operation table stood on the side of the access port and the vision cart was adjusted so that the surgeon next to the operation table, anesthesiologist and the nurse could see the monitor.
Three ports were used in Phase-1 patients and 3 ports and 1 access port in Phase 2 patients. The camera was inserted through the middle port. The interventions were performed with 3 ports and 1 access port in the first 20 cases in group Phase 3 and with 4 ports and 1 access port in the remaining patients (the latter group). In all interventions, a 30° camera was used in the up position during the access and in the down position during the resections. Regarding the locations of the ports in Phase 3 patients, the first port (camera, 12 mm) was inserted at the 7th intercostal space (ICS) on the posterior axillary line, the second port (12 mm) at the 6th ICS on the subscapular line (also used for the stapler), the third port (8 mm) at the 5th ICS on the anterior axillary line, and the fourth port at the 6th ICS with a distance of 3 cm to the paravertebral transverse process and the access port was inserted between the camera and anterior axillary line, third port at the 6th ICS with a 3 cm distance to the costodiaphragmatic symphysis. The access port was also used for the suction, retracting and removal of the materials [3].
During lobectomy, Maryland bipolar curved forceps (first arm) was inserted through the 2nd port, which was on the right side of the camera. Prograsper (second arm) was inserted through the 3rd port, which was on the left side of the camera and the second prograsper (third arm) was inserted through the 4th port, which was on the right or left side of the camera depending on the lateral positioning of the patient. In patients, who had an undiagnosed peripheral mass lesion, first 3 ports were opened at corresponding places. Lesions were resected and referred to frozen section for histopathologic analysis. In patients, in whom frozen section examination showed malignancy, the 4th and access ports were opened and robotic surgery was implemented.
The operative mortality was defined as the mortality due to any cause before the discharge within the first 30 days. In patients, who were converted to open surgery during the perioperative period, the results were evaluated according to the cause, postoperative morbidity/mortality, pathological analysis, and hospitalization duration.
The study protocol was approved by the local Institutional Review Board (Approval no: 2018/57), informed consent was obtained from each patient before the inclusion in the study.
Statistical Analysis
The data were collected and recorded on an Excel sheet (Microsoft Corporation, Seattle, WA). The mean, standard deviation, median, minimum, maximum values, frequency, and percentage were used for the descriptive statistics. The distribution of variables was checked with the Kolmogorov-Smirnov test. Mann-Whitney U test was used for the comparison of quantitative data. Chi-Square test was used for the comparison of qualitative data. SPSS 22.0 software package was used for statistical analyses. The statistical significance level was considered as p < 0.05.
Results
63 patients (26 males (41.3%) and 37 females (58.7%)) with a mean age of 55.4 ± 16.6 years had undergone RATS. The mean lesion size was 3.8 ± 1.2. Regarding all patients, the docking time, console time, operation time and length of hospitalization were 19.8 ± 6.5, 105.0 ± 64.4, 137.5 ± 73.4, and 6.2 ± 3.3 respectively. The robotic surgery-training program was designed in three phases. The age, sex, lesion side, lesion dimension, docking time, console time, and operation time, number of switches to the open surgery, complications, length of hospitalization and mortality rates of patients in all three groups were listed in table 1.Table 1: Demographic data, perioperative and postoperative results.
Regarding the 12 cases in group Phase 1, 5 patients underwent pericardial cyst excision, 2 patients pericardial window surgery, 2 patients sympathetic chain removal, 2 patients Schwannoma resection, and 1 patient underwent excision of the esophageal duplication cyst. The cystic lesions were excised totally after the aspiration of the cyst content. There was no need for VATS or thoracotomy in patients in group Phase 1. No mortality was encountered in the first 30 days after the surgery and no recurrence emerged during the long-term follow-up.
The 16 cases, who were included in group Phase 2, did not show a significant difference regarding the age, sex, and lesion size compared to group Phase 1 (p < 0.05). Three patients underwent thymothymectomy due to the thymoma, 3 patients bullectomy and parietal pleurectomy due to the bullous emphysema, 3 patients enucleation due to the hamartoma, 3 patients cystotomy and capitonnage due to the pulmonary cyst hydatid and 4 patients wedge resection due to the solitary pulmonary nodule. In cyst hydatid cases, we aspirated first the cyst content and then the germinative membrane was removed with endobag (Endobag, Covidien, Mansfield, MA, USA). The docking time was significantly shorter in this group compared to group Phase 1. However, the console time and operation time were significantly longer compared to group Phase 1 (p < 0.05). Three patients (18.75%) had a prolonged air leak, which was controlled with conservative treatment in two cases. One of the cyst hydatid cases applied with air leak (pneumothorax) after the discharge and underwent minithoracotomy. The bronchial leak was closed and the patient was discharged without any complication. No mortality or recurrence were observed in this group during the 30-day and long-term follow-up (Table 1).
In the group Phase 3 (n = 35); 29 patients (82.8%) had primary pulmonary malignancy, 3 patients (8.5%) metastatic lung carcinoma and 3 patients (8.5%) benign lesions. All patients underwent lobectomy. In patients with primary lung carcinoma, mediastinal lymph node dissection was added to the intervention. R0 resection was achieved in all cases with a primary lung tumor and all visible N2 lymph nodes were completely removed. Although the docking time was significantly shorter in Phase 3 compared to Phase 1 and Phase 2 cases, the console time and operation time was significantly longer than both of groups (p < 0.05) (Table 1).
The lobectomy cases, who targeted group Phase 3, were scheduled for RATS and underwent a much more detailed examination compared to the other two groups. The mean docking time, console time and operation time after the first 20 Phase 3 patients were significantly shorter than the first 20 patients (p < 0.05) (Figure 1).
![]() Click Here to Zoom |
Figure 1: Docking time of first 20 and latter cases (a), console time of first 20 and latter cases (b), operation time of first 20 and latter cases (c). |
Therefore, the first 20 cases were accepted as the learning curve (Table 2). There was no significant difference between the patients in the learning curve group and the remaining patients in the latter group regarding the demographic characteristics (p > 0.05). The lobectomy distribution of both groups is summarized in Table 3. In the lobectomy group, in 3 patients, the intervention was converted to open surgery during the perioperative period. The reason of the conversion was pulmonary artery bleeding (right upper lobe anterior segment artery and left lower lobe common basal segment pulmonary artery) in two cases and device dysfunction (stapler locked during the bronchial division in the right upper lobe). Hemorrhage was observed in one patient in each group. The mean hospitalization time in the ICU was 1 day (0-2 days). There was no significant difference between the learning curve group and the latter group regarding the conversion to thoracotomy (p > 0.05).
Table 2: Preoperative and postoperative data of learning curve and latter patients.
Table 3: The distribution of lobectomies and pathological classification.
Considering the whole Phase 3 lobectomy group, 11 patients (31.42%) had co-morbidities; 5 patients (14.28%) had prolonged air leak, 4 patients (11.42%) atrial fibrillation, and 2 patients (5.71%) pneumonia. The air leak was treated with conservative methods; in one case, it was brought under control with minithoracotomy on the 14th postoperative day. Three of 4 patients, who developed atrial fibrillation, was converted to the sinusoidal rhythm with amiodarone infusion and one patient with cardioversion. Patients with pneumonia were treated with antibiotics.
The pulmonary malignancy was the most common result in the pathological examination in lobectomy group (n = 32; 91.4%). Considering 29 patients with primary lung carcinoma, 17 of them had adenocarcinoma (58.62%), 8 squamous cell carcinoma (27.58%), 2 patients small cell carcinoma (6.89%) and 2 patients carcinoid tumor (6.89%) (Table 3). The pathological staging was as follows: 12 cases Stage I (IA n = 6, IB n = 6), 14 cases Stage II (IIA n = 8, IIB n = 6) and 3 cases Stage IIIA (n = 3).
All patients were examined with complete blood count, biochemical analysis, ECG, and posteroanterior chest x-ray examination on postoperative days 10, 30 and 90, and then at 3-month intervals. The mean follow-up duration was 28.4 months (2-56 months). No mortality was observed during the short-term follow up (30-90 days). One patient (2.85%) with metastatic lung carcinoma (colon adenocarcinoma) died in the 6th month after surgery in the Phase 3. The remaining cases are under follow-up and are disease-free.
Discussion
The robotic surgery becomes more popular from day to day and its spectrum becomes wider. Depending on the shorter hospitalization and lower complication rates and technological development in the robotic arms, robotic surgery became preferable against VATS and thoracotomy [4]. Therefore, efforts to develop RATS training programs are steadily increasing.There are articles in the literature, which indicate that robotic surgery training should have 3 phases. Ricciardi et al. [1] recommended that a simulator should be used in the first phase. The observation of the interventions implemented by an experienced surgeon, training on animals and cadavers should be considered in the second phase and in the third phase, the candidate should perform robotic surgery starting from simple cases with a dual console if available, if not under the supervision of a proctor. Some studies had divided three phases as 1st, 2nd and 3rd phase operations [5-7]. Like the recommendations in the literature, we planned our training in three phases after a lobectomy practice period on pigs [6-8].
In group Phase 1, the absence of complication and mortality and short hospitalization time was consistent with the literature [9]. In group Phase 2, we selected 2nd level pathological conditions similar to the literature [6-8]. In the Phase 2 patients, the rate of air leak (n = 3) was also similar to the results in the literature [10]. In the third and targeted last phase, who were planned for lobectomy operation, were included [7,10-12]. The mean docking time in all groups was consistent with the literature [11]. In the Phase 3 group, it is observed that docking time became significantly shorter along with the increase in experience. The significantly longer operation time in Phase 3 patients compared to Phase 1 and 2 patients was related to the complicated cases. Nevertheless, the results were consistent with the results in the literature (Table 4).
In group Phase 3, the first 20 cases were accepted as the lobectomy learning curve, as the docking time, console time and operation time were shortened after these cases. In various studies, the investigators reported that the operation time for lobectomy was between 104 and 226 minutes after the learning curve. The same time was 136.9 minutes in our study, which was consistent with the results of the published studies [13-14]. The curve representing the operation time of the first 20 patients showed a statistically significant decline (Figure 4).
![]() Click Here to Zoom |
Figure 2: Learning curve. |
The learning curve case number was 18 in the study, which was conducted by Veronesi et al. [15] (n=91), depending on operation time and hospitalization time and 14 in the study of Toker et al. [11] (n=102) depending on the docking time, console time and operation time. Huang et al. [16] conducted a study with 389 cases and reported the first 20 cases as the learning curve depending on the decrease in the complication and mortality rates. Melfi et al. [12] suggested that at least 20 cases were needed. It was reported that the general assumption was between 14-21 cases [17-19].
In some studies, the interventions were performed with 3 arms [14]. Although most of the RATS surgeons reported that they were using 3 arms and 1 access arm, Cerfolio et al. [20] recommended using of 4 arms. We used 3 arms and 1 access arm in our first 20 learning curve cases but then we switched to 4 arms and 1 access arm technique. We might suggest that the Complete Portal Robotic Lobectomy-4 (CPRL-4) 4-arm method enabled a better exposure with the CO2 insufflation, less microhemorrhage and consequently a shorter operation time. However, there are also studies, which reported that the complication rate was not decreased and it had no superiority over 3-arm technique or VATS [22].
Although we switched to thoracotomy in 3 cases (4.7%), all cases were in the lobectomy group. Regarding the literature, the conversion rates changed between 0% and 17%. There was no statistically significant difference between the learning curve group and the latter group in respect of conversion rates. The published studies showed that conversion rates declined in the learning curve and the latter groups [15,16,23]. Apparently, the relatively small number of the cases in the latter group was not adequate for an evaluation of the conversion rate.
In this study, we encountered complications in 14 patients (22.2%). 11 of these cases were in the lobectomy group. The reported complication rates in the literature were between 9% and 43%. The most common complications were prolonged air leak and arrhythmia. In some studies demonstrated that the most common complications were prolonged air leak and arrhythmia [8,16,25]. There was no statistically significant difference between the lobectomy learning curve group and the latter group regarding the complications. There are also other studies reporting that the console time and operation time were longer in the learning curve cases but the complication rates did not significantly differ [26].
None of the patients died during the 30-day and 90-day follow-up. One patient (1.5%) died during the long-term follow-up in all groups (mean duration 28.4 months). The 30-day and 90-day follow-up mortality rates in the literature (0%-3%) are comparable to our mortality rate. The mortality rate was 3% in the study of Toker et al. [11] during the 13-month follow-up. In the study of Veronesi et al. [22], none of the patients died at the early stage but the mortality rate became 3.29% (n = 3) during the 24-month (mean) follow-up. There are limited number of studies that reported 5-year follow-up periods [14]. Park et al. [21] conducted a multicenter study with 325 subjects and the disease-free survival rate was 80% (91% for Stage IA; 88% for Stage IB and 49% for Stage II). In a study of Yang et al. [27] (n = 175), the 5-year survival rate and the disease-free rates were 77% and 72% respectively. Our long-term follow-up results were consistent with the literature. Our 5-year follow-up results are not available yet.
The reported hospitalization duration was between 2 and 10 days in the literature. In our study, the hospitalization duration was 6.2 days. Although these results were within the limits of the interval in the literature, it is higher than the mean value. This result might depend on the absence of the outpatient drain follow-up [5,11].
VATS and robotic surgery were compared in several studies. Both of these techniques provide satisfying results in cancer surgery comparable to open surgery. VATS is usually performed with 2 incisions, while 4-5 incisions are used in RATS [24]. Although a larger number of incisions did not increase morbidity, it may aggravate postoperative pain. The operation time is generally longer in RATS, although recent studies showed that the operation time further shortened and became comparable to VATS [16]. In the same article, it was reported that the perioperative bleeding rate was lower in RATS. The intrathoracic maneuverability of the robotic surgery tools, a fixed camera with a 10x magnification capacity, 3D visualization, less pain, more comfortable esophagus surgery, and lymph node dissection are the advantages of RATS over VATS [19,25]. It was reported that the number of cases for the learning curve is smaller in RATS compared to VATS [28].
Veronesi et al. stated that 20 cases were enough for the learning curve in RATS but insufficient for VATS [15]. However, the high cost of RATS limits its advantages.
Besides on rapid training, RATS could be further supported with a 3-day surgery practice under the supervision of a mentor, promotion of the robotic system basics and tools within a program and multiple “tricks of trade” publishing. In addition, software packages such as “MIST-VR” and SI double-console can accelerate the training. Furthermore, consensus studies on robotic surgery training are ongoing.
Limitations of the Study
Our study on robotic training had a retrospective design. Randomized and controlled studies with prospective design are needed. The limited number of cases in the latter lobectomy group (cases after the learning curve), the absence of 5-year long-term follow-up results, quality of life, and cost analysis are the limitations of our study.
As a conclusion, regarding lobectomy, 20 cases might be considered as sufficient for robotic surgery training. Depending on the steep learning curve, robotic surgery is a safe, feasible, and minimally invasive surgical method with low complication and high success rates.
Declaration of conflicting interests
The authors declared no conflicts of interest with respect to the authorship and/or publication of this article.
Funding
The authors received no financial support for the research and/or authorship of this article.
Reference
1) Ricciardi S, Zirafa CC, Davini F, Melfi F. How to get the best from robotic thoracic surgery. J Thoracic Dis 2018; 10: S947-50.
2) Tchouta LN, Park HS, Boffa DJ, Blasberg JD, Detterbeck FC, Kim AW. Hospital volume and outcomes of robot-assisted lobectomies. Chest 2017; 151: 329-39.
3) Kocher GJ, Schmid RA, Melfi FM. Robotic lobectomy: tips, pitfalls and troubleshooting. Eur J Cardiothorac Surg 2014; 46: e136-8.
4) Kumar A, Asaf BB. Robotic thoracic surgery: the state of the art. J Minim Access Surg 2015; 11: 60-7.
5) White YN, Dedhia P, Bergeron EJ, Lin J, Chang AA, Reddy RM. Resident training in a new robotic thoracic surgery program. J Surg Res 2016; 201: 219-25.
6) Linsky PL, Wei B. Training in robotic thoracic surgery. J Vis Surgery 2018; 4: 1.
7) Cerfolio RJ, Bryant AS. How to teach robotic pulmonary resection. Semin Thorac Cardiovasc Surg 2013: 25: 76-82.
8) Eurico RJ, Santos SJ, Costa AR, Palma MF. The First 24 Robotic Surgeries of Hospital da Luz. Rev Port Cir Cardiothorac Vasc 2017; 24: 103.
9) Seder CW, Cassivi SD, Wigle DA. Navigating the pathway to robotic competency in general thoracic surgery. Innovations (Phila) 2013; 8: 184-9.
10) Yamashita SI, Yoshida Y, Iwasaki A. Robotic surgery for thoracic disease. Ann Thorac Cardiovascular Surg 2016; 22; 1-5.
11) Toker A, Özyurtkan MO, Kaba E, Ayalp K, Demirhan Ö, Uyumaz E. Robotic anatomic lung resections: the initial experience and description of learning in 102 cases. Surg Endosc 2016; 30: 676-83.
12) Melfi FM, Mussi A. Robotically assisted lobectomy: learning curve and complications. Thorac Surg Clin 2008; 18: 289-95.
13) Taniguchi Y, Nakamura H, Miwa K, Haruki T, Araki K, Takagi Y et al. Initial results of robotic surgery for primary lung cancer: feasibility, safety and learning curve. Yonago Acta Medica 2017; 60: 162-66.
14) Anderson CA, Hellan M, Falebella A, Lau CS, Grannis FW, Kernstine KH. Robotic-assisted lung resection for malignant disease. Innovations (Phila) 2007; 2: 254-8.
15) Veronesi G, Agoglia BG, Melfi F, Maisonneuve P, Bertolotti R, Bianchi PP et al. Experience with robotic lobectomy for lung cancer. Innovations (Phila) 2011; 6: 355-60.
16) Huang J, Li J, Li H, Lin H, Lu P, Luo Q. Continuous 389 cases of Da Vinci robot-assisted thoracoscopic lobectomy in treatment of non-small cell lung cancer: experience in Shanghai Chest Hospital. J Thorac Dis 2018; 10: 3776-82.
17) Gharagozloo F, Margolis M, Tempesta B, Strother E, Najam F. Robot-assisted lobectomy for early-stage lung cancer: report of 100 consecutive cases. Ann Thorac Surg 2009; 88: 380-4.
18) Meyer M, Gharagozloo F, Tempesta B, Margolis M, Strother E, Christenson D. The learning curve of robotic lobectomy. Internal J Med Robot 2012; 8: 448-52.
19) Lee BE, Korst RJ, Kletsman E, Rutledge JR. Transitioning from video-assisted thoracic surgical lobectomy to robotics for lung cancer: are there outcomes advantages? J Thorac Cardiovascular Surg 2014; 147: 724-9.
20) Cerfolio RJ, Bryant AS, Minnich DJ. Starting a robotic program in general thoracic surgery: why, how, and lessons learned. Ann Thorac Surg 2011; 91: 1729-37.
21) Park BJ, Melfi F, Mussi A, Maisonneuve P, Spaggiari L, Da Silva RK et al. Robotic lobectomy for non–small cell lung cancer (NSCLC): long-term oncologic results. J Thorac Cardiovascular Surg 2012; 143: 383-9.
22) Veronesi G, Galetta D, Maisonneuve P, Melfi F, Schmid RA, Borri A et al. Four-arm robotic lobectomy for the treatment of early-stage lung cancer. J Thorac Cardiovascular Surg 2010; 140: 19-25.
23) Gallagher SP, Abolhoda A, Kirkpatrick VE, Saffarzadeh AG, Thein MS, Wilson SE. Learning curve of robotic lobectomy for early-stage non-small cell lung cancer by a thoracic surgeon adept in open lobectomy. Innovations (Phila) 2018; 13: 321-7.
24) Louie BE, Farivar AS, Aye RW, Vallières E. Early experience with robotic lung resection results in similar operative outcomes and morbidity when compared with matched video-assisted thoracoscopic surgery cases. Ann Thorac Surg 2012; 93: 1598-605.
25) Toker A, Ayalp K, Uyumaz E, Kaba E, Demirhan Ö, Erus S. Robotic lung segmentectomy for malignant and benign lesions. J Thorac Dis 2014; 6: 937-42.
26) Özyurtkan MO, Kaba E, Toker A. What happens while learning robotic lobectomy for lung cancer? J Vis Surg 2017; 3: 27.
27) Yang HX, Woo KM, Sima CS, Bains MS, Adusumilli PS, Huang J et al. Long-term survival based on the surgical approach to lobectomy for clinical stage I non-small cell lung cancer: comparison of robotic, video assisted thoracic surgery, and thoracotomy lobectomy. Ann Surg 2017; 265: 431-37.